Whatever Happened to ‘the Big One’?
The quest to predict major earthquakes before they happen
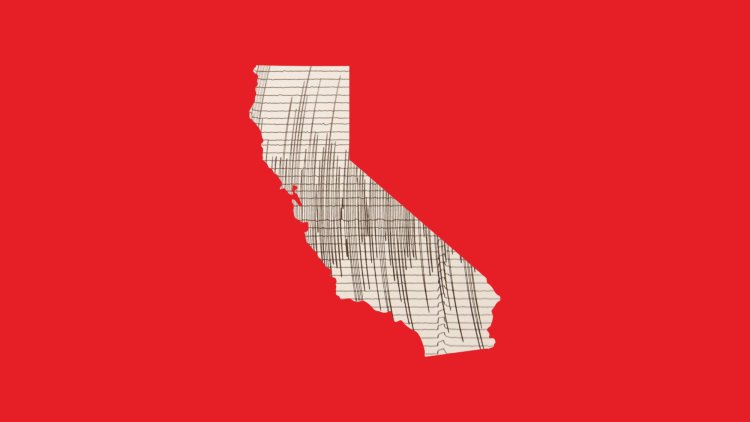
The three words were spelled out in block letters on the evening news, right next to an anchorman’s gelled coif: the big one. A map of Southern California hovered just below, bull’s-eye’d with red, concentric circles. I’m pretty sure it was 1988. The region was awash in warnings about the great earthquake to come. They were like something out of the Book of Isaiah. They lent an apocalyptic crackle to the sunbaked days. My generation hit school age too late to take part in atomic-attack drills, and too early for those that mimic school shootings, but we learned to duck and cover just the same.
In 1989, we saw a vision of our future. An earthquake hit the Bay Area, and for more than a week, the whole state was immersed in the imagery of seismic catastrophe. A freeway overpass snapped in half. Some of San Francisco’s pastel Victorians toppled over. A fire broke out in the Marina. The World Series—an event of great import and inevitability in the mind of a child—was halted. “The big one is supposed to be worse,” we whispered to ourselves, and to one another. In the psychogeography of Southern California, it lay sleeping like a monster deep beneath the Earth’s surface. At any moment, probably soon, it would wake up.
It still hasn’t. The San Andreas Fault formed about 30 million years ago, when the Pacific plate—the planet’s largest—began grinding against the North American plate. Sometimes, the plates snag. Tension builds until they release with a lurch that sends energy in all directions. The section of the San Andreas that runs alongside Los Angeles hasn’t had a fearsome quake for more than three centuries. Paleoseismologists expect big ones to occur there every 150 to 200 years, Greg Beroza, a Stanford professor and a co-director of the Southern California Earthquake Center, told me: “We’re overdue.” Teams of scientists have been trying to improve on that chillingly vague forecast, he said, so that the quake’s arrival can be predicted days, weeks, or even months ahead of time—but there is no guarantee that they’ll succeed.
[From the January 1924 issue: A harrowing first-hand account of the Great Kanto earthquake]
At the turn of the 20th century, California was home to only a few seismographs, primarily inside domed observatories atop mountain peaks, where they made use of ultraprecise astronomers’ clocks. But after the United States and the Soviet Union agreed in 1963 to stop testing nukes aboveground, the Pentagon suddenly became very keen on funding new seismic sensors. Scientists have since spread more than 1,000 of them across California’s surface, in both big cities and wilderness areas. They pick up a lot of noise. East of Los Angeles, in the San Bernardino Mountains, they detect the clatter of rockfall. At construction sites downtown, they register the rumble of semitrucks and jackhammer pounding.
Algorithms sift through this noise at real-time data-analysis centers, searching for P-waves—the fast-moving ripples of seismic energy that first rush outward from a fault slip. These waves are a gentle announcement of the more ruinous S waves to come. When enough of them are detected, automated processes are set into motion. Millions of push alerts pop up on mobile-phone lock screens. Stop lights redden, and gas valves shut down. Metro cars pause instead of entering tunnels. “The speed of telecommunications is faster than seismic waves,” Beroza told me. “You only have seconds, but that’s enough time to get out ahead of the shaking.”
Scientists hope that new technologies can give us a longer warning window. If deep-learning algorithms were trained on enough seismic data, they might be able to spot sequences of activity that reliably precede earthquakes. So far, the forecasting track record of these techniques has underwhelmed, says Zachary Ross, a geophysicist at the California Institute of Technology. An analogous approach has had fabulous success in meteorology, he told me. AI-based methods can spot patterns in atmospheric data that help forecast storms, but they can’t yet guess when two plates along a fault might slip.
I was surprised to hear that the ethereal movements of wind and rain are easier to predict than great shifts in the hard subterranean realm of rock. Ross told me that meteorologists have better data. They’re constantly measuring the state of the atmosphere at high resolution, whereas seismologists are more constrained in the kind of data that they can collect, at least for now. Christopher Johnson, a research scientist at the Los Alamos National Laboratory, has been using a hydraulic press to push 10-centimeter blocks of granite together, until they slip past each other like tectonic plates. He measures the seismic energy that radiates outward, and is able to generate a lot of data, because these experiments can be repeated with new blocks in quick succession. But there are serious challenges in extrapolating from their results to actual earthquakes that operate on much larger scales of space and time. Fresh data are also pouring in from new kinds of sensors, including cheap ones that seismologists are deploying aboveground in temporary arrays along particularly active sections of faults. They’re also transforming existing fiber-optic networks into seismometers, by measuring changes to the way that laser light bounces around inside them when the ground shakes.
These new data sources are exciting, Ross said, but he doesn’t expect them to produce any breakthroughs in earthquake prediction. He said that emerging technologies have the same problem as existing ones: The sensors are all placed too far away from the action. California’s seismic sensors are at ground level, but the fault slips that trigger many of the state’s earthquakes start six to eight miles below the surface of the Earth. The resulting seismic waves radiate outward in a sphere. Some move downward through the thick molten mantle and core until they reach the planet’s opposite side. (“Anything over a 5 on the Richter scale is detected pretty much everywhere on Earth where there is a seismometer,” Ross told me.) Others rush up toward the surface directly above the fault. The problem is that no matter where these waves surface, they first have to pass through the last outer mile of crust, which is shot through with cracks, loose rock, and sloshing fluids. “Something like 90 percent of the energy of these waves gets absorbed just in the top mile or so,” Ross said. Seismologists can tell that an earthquake is happening, but they can’t observe its more subtle characteristics.
“Everything we do now is remote sensing,” Beroza told me. If we want clearer glimpses at earthquakes—and the potentially predictive seismic activity that precedes them—we have to place sensors underneath this top layer of crust. Japan’s seismic network is the envy of the world, in part because its scientists have wedged their sensors deeper into the planet. After the Kobe earthquake killed more than 6,000 people in 1995, the country’s political leaders encouraged data-sharing among seismologists and funded the drilling of boreholes all across the archipelago. Each one runs a few hundred feet deep.
It’s a good start. At that depth, the sensors encounter much less noise. But seismic waves are still distorted and weakened when they arrive at Japan’s borehole sensors. Ideally, they would be placed miles and miles down, where the quakes originate, but that part of the interior is as inaccessible as outer space, Beroza told me. Even putting the equipment a mile down would be very expensive. Any borehole that deep would be in constant danger of closing in on itself, given the extreme pressure. It might also fill up with corrosive liquids and gases. Still, this kind of drilling has been done in a few one-off projects. If it were successful, sensors could be lowered beneath the crust’s uppermost layer, and they’d be able to record aspects of an earthquake that can’t be observed at the surface.
“The most persistent hope is that there’s some signal in smaller earthquake activity that would presage larger earthquakes,” Beroza said. But scientists might also pick up new kinds of seismic waves, or new patterns of activity. Ross told me that any breakthrough in prediction would most likely arise from a signal that we’ve never seen before and don’t expect. But even if we spend decades listening closely to the great monster that lies sleeping beneath California, we may never hear any telltale pattern of snoring that suggests it is about to rouse itself. We may forever be vulnerable to big ones.
I had hoped for better news. I no longer live in Southern California, but I remain tethered to it, spiritually and through family. Fear of the big one is a shared psychic experience for those who grew up in the region. I still remember worrying over it with friends on the playground and late at night during sleepovers. Like the Night Stalker, it seemed to be a hyperlocal generational concern, an object of anxiety that was uniquely ours. But we have passed it down. All these years later, the forecast hasn’t changed: Experts are still giving even odds that a great quake will shake the massed towers of downtown Los Angeles and its surrounding environs, with great violence, sometime in the next 30 years. When my young niece and nephew go to school, they too may be taught to fear the big one. Part of growing up in California, or anywhere, is learning that science has limits. Nature can never be fully known or tamed.
What's Your Reaction?
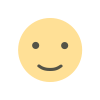





